With rising water quality standards, it is of great importance to develop processes that can efficiently remove recalcitrant pollutants which are resistant to conventional water treatment methods. While developing such processes, the energy efficiency and the economy of the process plays a key role as implementation of these process on large scale can only happen if they are economically feasible. And therefore, the use of renewable energies in the removal of contaminants looks like the way forward.
Advanced Oxidation Processes (AOPs) are used to achieve complete mineralization of the pollutants by the production of hydroxyl radicals. Most common methods include ozonation, UV/H2O2, sonolysis, photocatalysis etc. Photocatalysis however is a process to remove pollutants and microorganisms with the use of UV and visible light. The attractive feature of this process is the use of solar energy which makes the process sustainable and clean. A lot of research has been done to evaluate the use of photocatalysis for various pollutants under diverse operating conditions. Many other research focus on the development of cheaper novel photocatalysts to further reduce the cost of the process. However, it has been observed that the efficiency of the photocatalytic process reduces drastically due to the recombination of the charge carriers.
Photoelectrocatalysis (PEC) is a promising new technology to overcome the limitations of the conventional photocatalysis. This involves the immobilization of the photocatalyst on a conductive substrate which is used also as an electrode. The electric bias separates the electron-hole pairs and prevents their recombination. The positively charged holes migrate to the photocatalyst surface where they directly oxidize the organic pollutant and the negatively charged electrons migrate to the counter electrode through the external circuit [1]. As compared to photocatalysis, PEC increases the efficiency of the process and produces higher number of holes [2]. The immobilization of the photocatalyst is a worthwhile advantage of the PEC process. By doing so, the need for catalyst recovery systems is eliminated. The research in this field has been growing as more and more applications of PEC in water treatment have been discovered. A simple search on Scopus for “photoelectrocatalysis” revealed a total of 1,317 published work on the topic within the last 10 years (2011-2021).
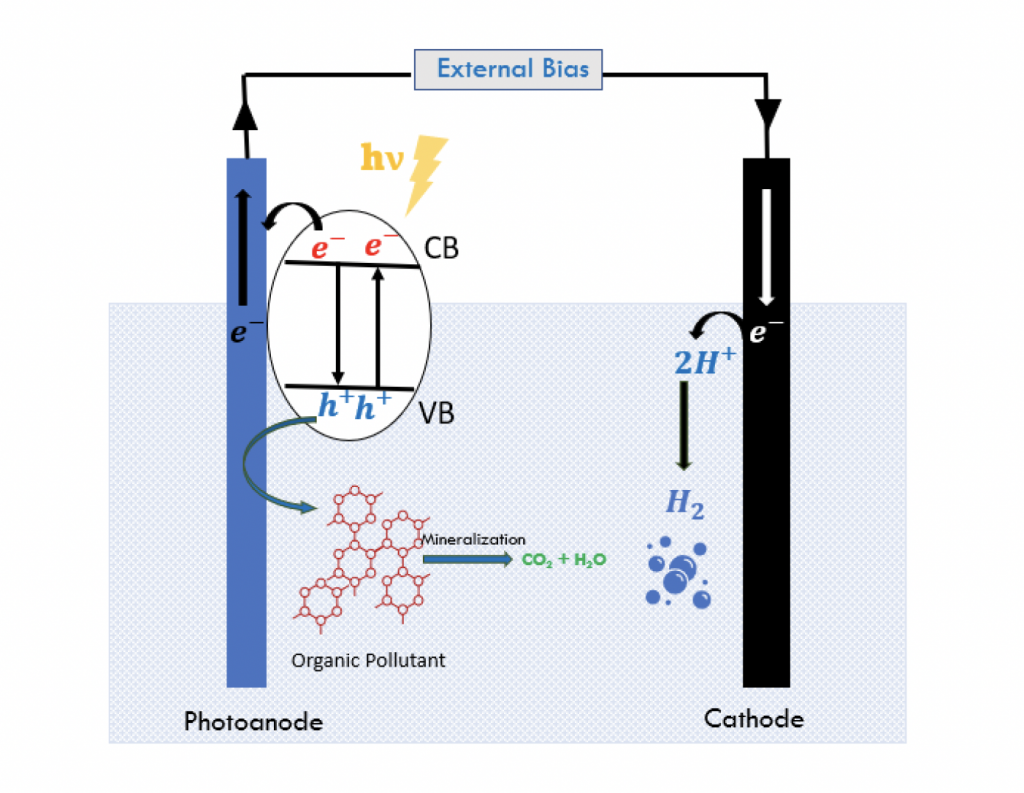
Figure 1: Schematic of PEC process
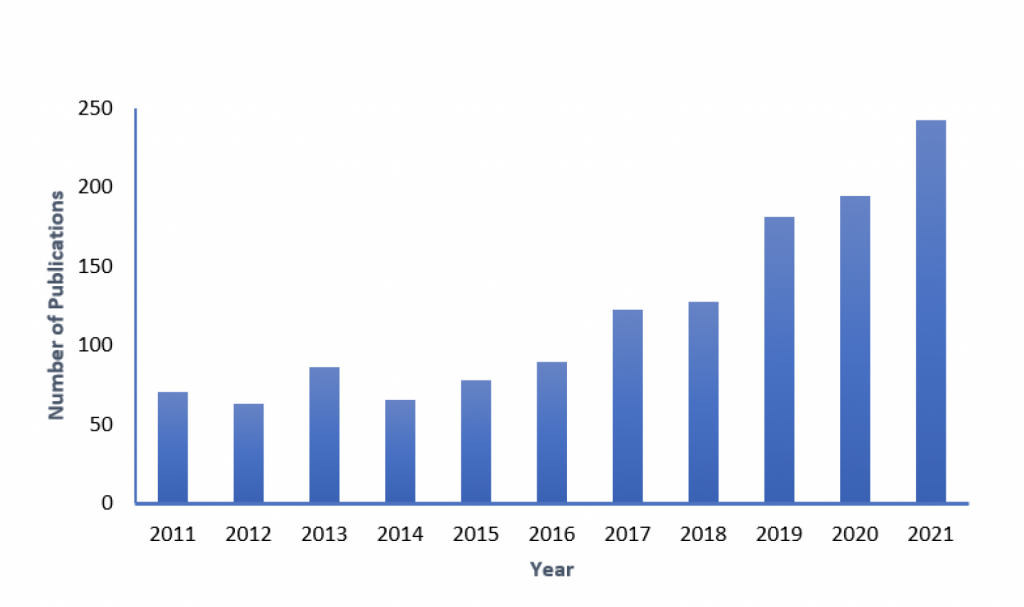
Figure 2: Number of publications on PEC
The PEC process has also been successfully used to generate hydrogen while simultaneously removing pollutants from wastewater. The holes generated are used to oxidize the pollutants at the anode while the electrons at the cathode are used to produce hydrogen by reduction reactions. In this configuration, the benefit of energy recovery as hydrogen fuel is combined with the reduction of environmental pollution in the water ecosystem [3]. Many reactor configurations have been developed for the PEC process however, there are not many research works on the effect of reactor configurations on this process. One of the most used reactor configurations to produce H2 is the H-cell where the cathode and the anode compartment are separated and only one pollutant is used in the anode compartment to generate hydrogen [4]. The use of real wastewater samples to produce hydrogen remains a challenge and only limited work has been done with this regard. By minor modifications, such system can also be used to generate hydrogen peroxide which later can be coupled with other AOPs to generated hydroxyl radicals [5].
One of the important aspects in a PEC process is the choice of the semiconductor material that is used as a photocatalyst because the efficiency of the process is greatly affected by the material. TiO2 is a widely used photocatalyst due to its high stability and low environmental impacts. Apart from TiO2 other semiconductors like ZnO, WO3, BiVO4, Fe2O3 etc. have been widely tested in PEC systems. However, each material has a certain limitation of its own, for example, the insensitivity of TiO2 to visible light, the stability issues of ZnO in aqueous medium and the need for threshold bias for BiVO4. Therefore, the right choice of material depending on the purpose of the process becomes very vital.
Several variables need to be considered while designing PEC reactors for real-life applications. The important ones include the mass transport, the distance between the electrodes, the surface to volume rations and the oxygen supply for the generation of the reactive oxygen species (ROS) [3]. The optimization of such reactors needs to be done using simulation softwares due to complex coupled mechanisms within the reactor. The use of real solar irradiation further complicates the process due to the large variation in the irradiation depending on the time of the day and the seasonal changes which in turn leads to changes in the reaction times. The next challenge arises in the scale up and industrial application of the PEC process. The use of ultrapure water and addition of huge quantities of electrolytes limits the scale up of the process. For successful scale-up of the PEC systems more work needs to be done to study the effect of real water matrices (domestic or industrial) in these systems. Unless the effect of multiple components such as carbonates, organics, chlorine etc. present in the real wastewater is evaluated, the scale-up of such process is not at hand.
With proper selection of the electrode materials, careful optimization of reaction parameters and design, the PEC process can be made more effective. This process is no doubt more efficient as compared to conventional photocatalysis as it helps in fast transport of the generated charge carriers and prevents recombination. With this as an advantage and the use of clean solar energy, this process could be a sustainable technology when combined with generation of hydrogen simultaneously. And as far as scale-up is concerned, research on PEC process needs to branch out into synthetic wastewater and then into real wastewater to evaluate their use as a sustainable clean technology.
[1] M. Coha, G. Farinelli, A. Tiraferri, M. Minella, D. Vione, Advanced oxidation processes in the removal of organic substances from produced water: Potential, configurations, and research needs, Chem. Eng. J. 414 (2021) 128668. https://doi.org/10.1016/j.cej.2021.128668.
[2] S. Garcia-Segura, E. Brillas, Applied photoelectrocatalysis on the degradation of organic pollutants in wastewaters, J. Photochem. Photobiol. C. 31 (2017) 1–35. https://doi.org/10.1016/j.jphotochemrev.2017.01.005.
[3] P. Fernandez-Ibanez, S. McMichael, A. Rioja Cabanillas, S. Alkharabsheh, A. Tolosana Moranchel, J.A. Byrne, New trends on photoelectrocatalysis (PEC): nanomaterials, wastewater treatment and hydrogen generation, Curr. Opin. Chem. Eng. 34 (2021) 100725. https://doi.org/10.1016/j.coche.2021.100725.
[4] A. Rioja-Cabanillas, D. Valdesueiro, P. Fernández-Ibáñez, J.A. Byrne, Hydrogen from wastewater by photocatalytic and photoelectrochemical treatment, J. Phys. Energy. 3 (2020) 012006. https://doi.org/10.1088/2515-7655/abceab.
[5] R. Dhawle, Z. Frontistis, D. Mantzavinos, P. Lianos, Production of hydrogen peroxide with a photocatalytic fuel cell and its application to UV/H2O2 degradation of dyes, Adv. Chem. Eng. 6 (2021) 100109. https://doi.org/10.1016/j.ceja.2021.100109.
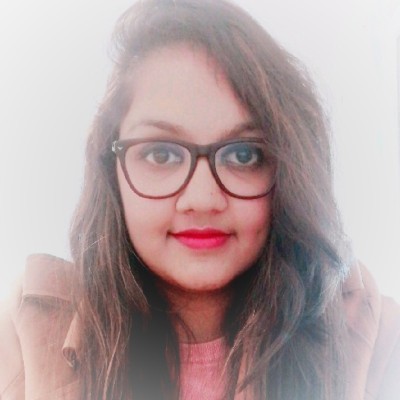
Rebecca is a Chemical Engineer from Bangalore, India. Since her school days she was fascinated by science and technology. She specialized in biology and chemistry during her high-school and then went ahead to get her Bachelor of Engineering degree in Chemical Engineering from the MVJ College of Engineering in Bangalore, India.
In 2017, she moved to Toulouse, France and pursued her MSc in Fluids Engineering for Industrial Process from the The Institut National des Sciences Appliquées de Toulouse (INSA Toulouse). The program focused on the physical analysis and modeling of coupled transfer mechanisms in multiphase flows. She had the opportunity to learn and use multiple CFD softwares during the Master’s program. She did her research internship at the Laboratory of Chemical Engineering, Toulouse. She worked on the “Modelling Of A Homogenous Photo-Catalytic Reactor For The Degradation Of Organic Pollutants Using AOPs”. This project kindled her interest in the field of advanced oxidation processes for water treatment.
Currently, Rebecca is a Marie Curie Early Stage Researcher (ESR6) in the InnovEOX project at the Chemical Engineering department of the University of Patras, Greece. She is working on “Hybrid Photoelectrochemical Processes” under the supervision of Prof. Dionisios Mantzavinos.