Low temperature plasma generated at atmospheric pressure has several applications in different fields such as agriculture and water treatment [1], [2]. It has been shown that cold plasma generated in or in-contact with water can degrade micro-pollutants present in wastewater. Plasma generated in air at atmospheric pressure produces a reactive Oxygen and Nitrogen species such as N, O, OH etc. due to the generation of fast electrons. These species have the ability to degrade organic molecules present in water without producing secondary waste products [3], [4].
Atmospheric pressure plasma has been found to be very effective compared to other advanced oxidation processes, there remains quite a challenge as to the type of reactor and power source that can provide the highest efficiency. In this aspect, it has been shown that the most efficient systems used pulsed power to generate plasma [5].
When microsecond duration high voltage pulses are applied to the electrode of the reactor, the electrons are accelerated due to the presence of high intensity electric field [6], [7]. These electrons produce the reactive radical species. Moreover, the system is off between short pulses which helps the system to cool down unlike DC generated plasma.
To study these short pulses, it is important to understand about the pulse power sources that are used to generate these pulses. A pulsed power source can be designed in different ways depending on the application and the load. Some examples of the pulsed power source include single line pulse source, Blumlein line pulse source, transmission line transformer, Marx generator and magnetic pulse compression pulse source. A general pulsed power source consists of three blocks: an energy storage element, a high-voltage switch and a load.
A high-voltage switch is the most important component of the power source as it should be able to handle a very high voltage and current. There are two types of switches used in sources: gas switches [8] and solid-state switches [9]. The advantage of gas switches such as spark gaps is that they can handle high voltages. However, the disadvantage is that they require a lot of maintenance and they have a low repetition rate. Solid state switches such as semiconductor switches can typically operate at high repetition rates. However, owing to their limited voltage and current handling capacity, series and parallel arrays of switches are required. This makes the power supply circuit more complicated and difficult to trigger. Different types of semiconductor switches have been used such as step recovery diodes, avalanche transistors, MOSFETs and IGBTs.
The second component of the power source is the energy storage element. Energy storage elements are usually capacitors or transmission lines. When employing a transmission line, it becomes possible to achieve low rise times. However, matching the load can often prove to be difficult. This can lead to reflections at the load and a subsequent decrease in voltage. On the other hand, when capacitive energy storage is used, there is always exponential decline in voltage. Lastly, most pulse sources are designed depending on the impedance of the load, whether it is resistive, capacitive, or inductive.
The third component of the pulse source is the load that is dynamic in case of plasma. The plasma is entirely capacitive at first. Then it becomes resistive as the plasma starts to form. The designed pulsed source should be able to handle both capacitive and resistive loads [10].
Another point to consider is the polarity of the pulses generated. Negative and positive voltage pulses offer distinct advantages in plasma applications. Negative voltage pulses allow for higher electron densities and improved plasma uniformity. The negative polarity enables precise control over plasma characteristics, making it suitable for applications that require consistent plasma conditions. On the other hand, positive voltage pulses can achieve higher plasma temperatures and increased ionization efficiency, making them advantageous for processes that require intense heat and efficient ionization.
In conclusion, low temperature atmospheric pressure plasma has emerged as an efficient method for water treatment. Cold plasma has demonstrated its efficacy in degrading micro-pollutants in wastewater without generating secondary waste products. Pulsed power systems have been identified as the most efficient in generating plasma, offering control over plasma characteristics. Understanding pulse power sources and their components, such as high-voltage switches and energy storage elements, is crucial for designing efficient plasma systems. The choice between negative and positive voltage pulses depends on specific application requirements, with negative pulses offering higher electron densities, while positive pulses enable higher plasma temperatures. Atmospheric pressure plasma holds great promise for future advancements in various fields, presenting opportunities for environmentally friendly and effective technologies.
References:
[1] M. Laroussi, ‘Cold Plasma in Medicine and Healthcare: The New Frontier in Low Temperature Plasma Applications’, Frontiers in Physics, vol. 8. Frontiers Media S.A., Mar. 20, 2020. doi: 10.3389/fphy.2020.00074. [2] A. Barjasteh, Z. Dehghani, P. Lamichhane, N. Kaushik, E. H. Choi, and N. K. Kaushik, ‘Recent progress in applications of non-thermal plasma for water purification, bio-sterilization, and decontamination’, Applied Sciences (Switzerland), vol. 11, no. 8. MDPI AG, Apr. 02, 2021. doi: 10.3390/app11083372. [3] I. Oller, S. Malato, and J. A. Sánchez-Pérez, ‘Combination of Advanced Oxidation Processes and biological treatments for wastewater decontamination-A review’, Science of the Total Environment, vol. 409, no. 20. pp. 4141–4166, Sep. 15, 2011. doi: 10.1016/j.scitotenv.2010.08.061. [4] A. Kumar, N. Škoro, W. Gernjak, and N. Puač, ‘Cold atmospheric plasma technology for removal of organic micropollutants from wastewater—a review’, European Physical Journal D, vol. 75, no. 11, Nov. 2021, doi: 10.1140/epjd/s10053-021-00283-5. [5] M. A. Malik, ‘Water purification by plasmas: Which reactors are most energy efficient?’, Plasma Chemistry and Plasma Processing, vol. 30, no. 1, pp. 21–31, Feb. 2010, doi: 10.1007/s11090-009-9202-2. [6] R. Ono, Y. Nakagawa, and T. Oda, ‘Effect of pulse width on the production of radicals and excited species in a pulsed positive corona discharge’, J Phys D Appl Phys, vol. 44, no. 48, Dec. 2011, doi: 10.1088/0022-3727/44/48/485201. [7] E. J. M. van Heesch, G. J. J. Winands, and A. J. M. Pemen, ‘Evaluation of pulsed streamer corona experiments to determine the O * radical yield’, J Phys D Appl Phys, vol. 41, no. 23, 2008, doi: 10.1088/0022-3727/41/23/234015. [8] A. A. Neuber, Y. J. Chen, J. C. Dickens, and M. Kristiansen, ‘A compact, repetitive, 500KV, 500 J, Marx generator’, in Digest of Technical Papers-IEEE International Pulsed Power Conference, 2007, pp. 1203–1206. doi: 10.1109/PPC.2005.300570. [9] H. Canacsinh, L. M. Redondo, and J. F. Silva, ‘Marx-type solid-state bipolar modulator topologies: Performance comparison’, IEEE Transactions on Plasma Science, vol. 40, no. 10 PART 1, pp. 2603–2610, 2012, doi: 10.1109/TPS.2012.2190944. [10] T. Huiskamp, ‘Nanosecond pulsed streamer discharges Part I: Generation, source-plasma interaction and energy-efficiency optimization’, Plasma Sources Science and Technology, vol. 29, no. 2. Institute of Physics Publishing, Feb. 11, 2020. doi: 10.1088/1361-6595/ab53c5.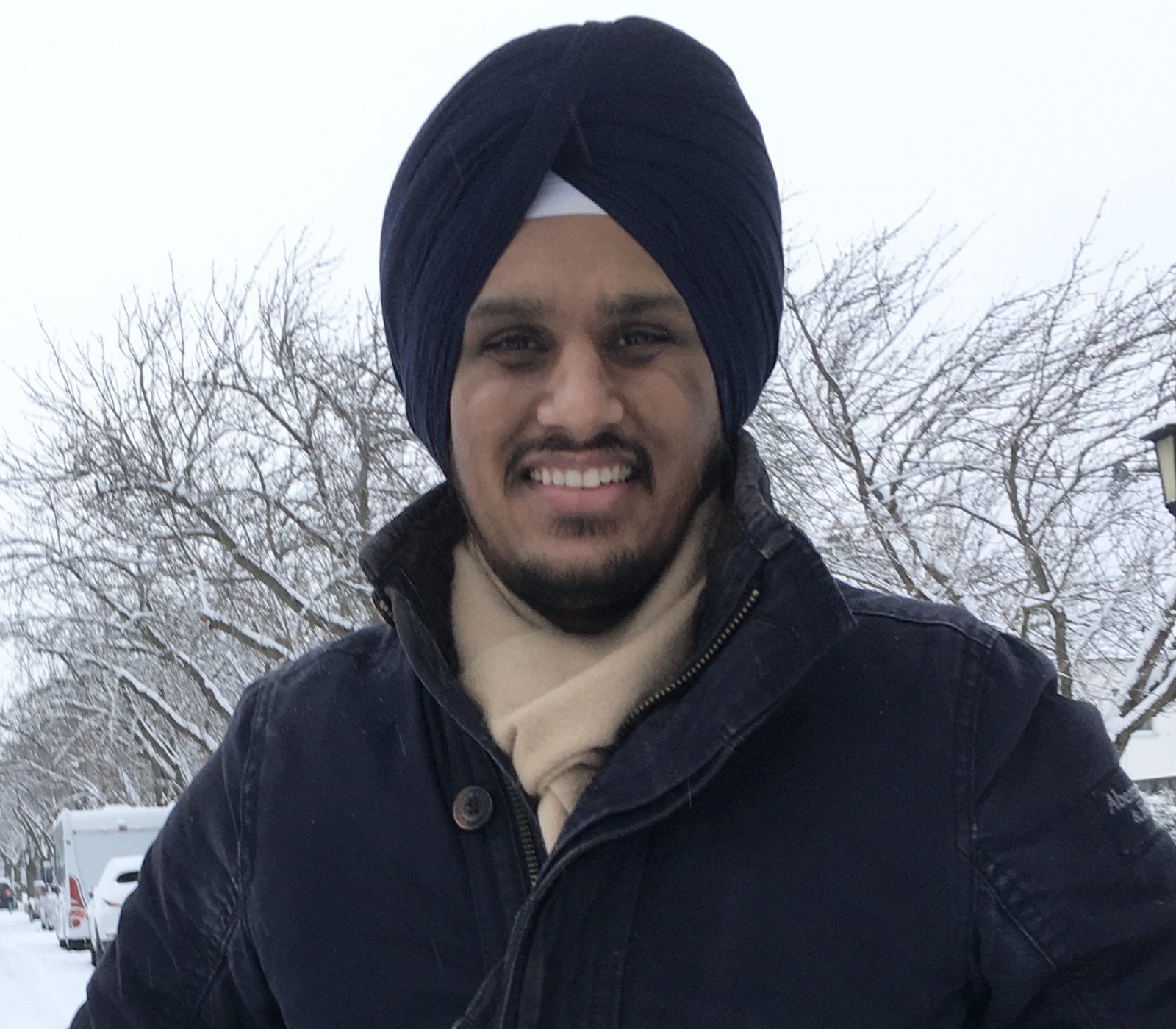
Gagandeep is an Electrical and Electronics Engineer from India. e Early Stage Researcher (ESR2) in the InnovEOX project at the Electrical Engineering department of the University of York, United Kingdom. He is working on the manipulation of plasma species generation in an advanced oxidation process under the supervision of Prof. James Walsh.